Author: Chief Scientist of Hengxin Dimension
Copyright Notice: Unauthorized media reproduction and excerpting in any form are strictly prohibited. If you need to reprint, please contact Shenzhen Weiwei Technology
Preface/PREFACE
At the beginning of this month, the Royal Swedish Academy of Sciences announced on its website that the 2023 Nobel Prize in Physics will be awarded to three scientists, Pierre Agostini, Ferenc Krausz, and Anne L'Huillier (from the United States, Germany, and Sweden, respectively), in recognition of their outstanding contributions in the field of attosecond science research. For those who have been engaged in and concerned about ultrafast laser optics research for a long time, this is indeed a very inspiring and exciting thing.
[1] The specific reason for the award is the experimental method of generating attosecond light pulses for studying the electron dynamics of matter.
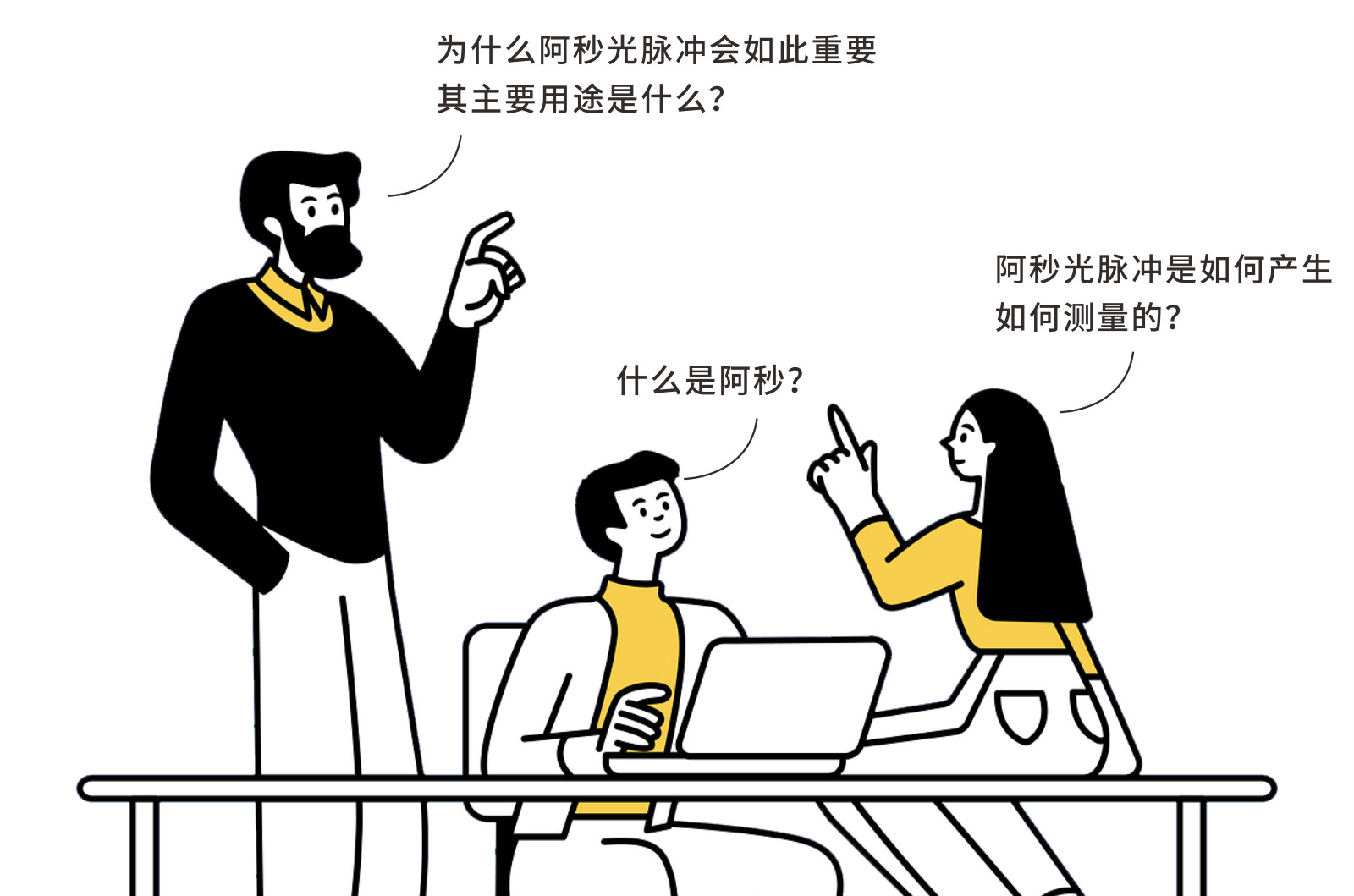
What is a second? We know that time is the most basic scale for measuring the movement and changes of all things in the world. Meanwhile, it is also the most commonly used physical quantity in physical equations. The time unit known to people in daily life is the second, which is the time when a person's heart rate is 60 beats per minute. In the direction where time becomes shorter, one thousandth of a second is one millisecond; One thousandth of a millisecond is one microsecond; One thousandth of a microsecond is one nanosecond; Milliseconds (10-3s), microseconds (10-6s), and nanoseconds (10-9s) are commonly encountered time scales in dynamic testing in the technical field. If we take 1 nanosecond as the base point and further subdivide it, then one thousandth of 1 nanosecond is 1 picosecond; One thousandth of a picosecond is one femtosecond; One thousandth of a femtosecond is one attosecond.
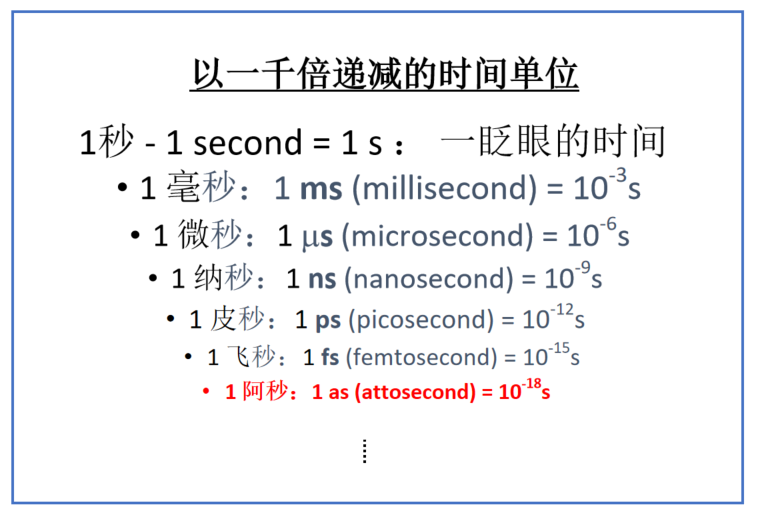
If 1 second is divided by 1000, it takes six consecutive divisions to reach 1 attosecond (10-18 seconds). So it can be said that a second is a very, very... very brief (very six times) moment. In such a short moment, we humans cannot feel it at all. Therefore, a rough concept can only be obtained through relative comparison methods: for example, 1 attosecond (10-18s) relative to 1 second is approximately equivalent to the lifespan of 1 second relative to the universe (1018s) [2]. In fact, picoseconds (10-12s) and femtoseconds (10-15s) are commonly encountered time measures in the research of ultrafast phenomena and the application of ultra short pulse laser processing today. Only in the past 20 years have scientists truly begun to step into the attosecond time scale from experiments.
[2] According to the currently known lifespan of the universe, which is 13.8 billion years, converted to seconds, it is 0.44x1018 seconds.
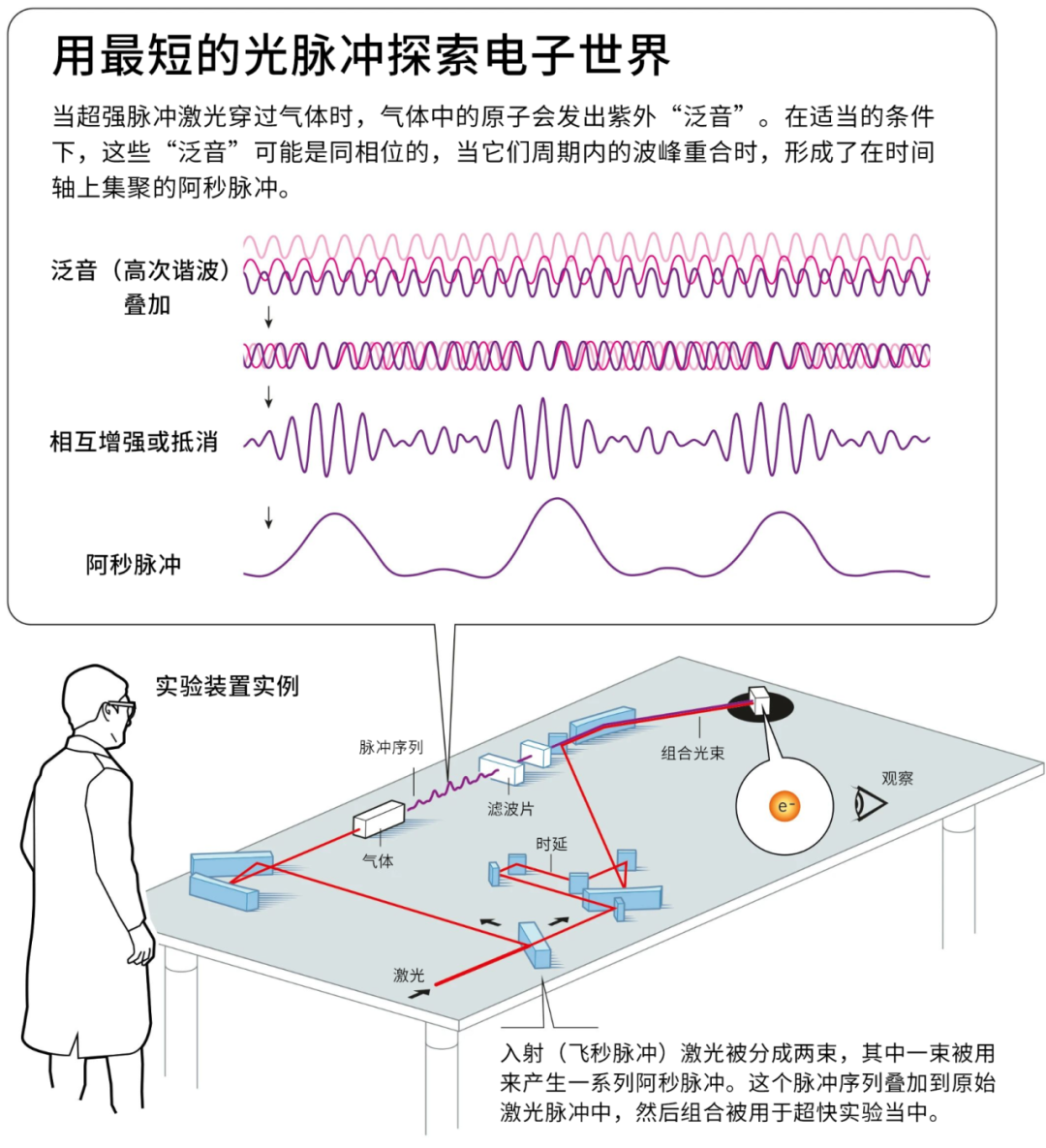
Translated from "popular physicsprize2023", THE ROYAL SWEDISH ACADEMY OF SCIENCES ★ WWW.KVA.SE
After understanding what attosecond and attosecond light pulses are, the next question naturally becomes: how are attosecond light pulses generated and measured? Here, we may borrow a picture from the introductory article of the Science Popularization Edition of the Nobel Prize in Physics published by the Nobel Prize Committee on its website. This diagram will briefly illustrate the basic principle and core experimental setup for generating and measuring attosecond light pulses. In short, the generation of attosecond light pulses is generated by the action of high-quality and high-intensity few period femtosecond light pulses on inert gas targets. The important scientific discoveries and key technologies involved include high-order harmonic generation, optoelectronic measurement techniques with attosecond response time, and the generation of a single attosecond optical pulse through XUV extreme ultraviolet light filtering. It is not difficult to imagine that if accurate and reliable measurements cannot be achieved in the experiment, the generation of attosecond light pulses will become a myth. Therefore, the generation of attosecond light pulses is closely related and inseparable from the measurement of attosecond light pulses [5].
[3] An attosecond light pulse can be understood as a flash with a duration of at the attosecond level.
[4] The upper box in the figure illustrates that the coherent superposition of high-order harmonics generated by femtosecond laser in gas forms attosecond optical pulses.
[5] In fact, it is possible that attosecond light pulses were already generated in some early experiments on high-order harmonic generation, but at that time there was neither a theoretical model nor experimental means to verify its existence.
High order harmonic generation [6]
When ultra short and ultra strong laser pulses are focused on atomic gases, high-order harmonics are generated (see schematic diagram below). This phenomenon was first discovered by Anne L'Huillier, one of the Nobel laureates of this year, and her colleagues during her postdoctoral research in France in 1987 (J. Phys. B: At Mol. Opt. Phys. 21, L31 (1988)). In the following two to three decades, with the continuous development of laser and testing technology, many international research teams have conducted extensive and in-depth research on the generation of high-order harmonics in gases [7]. The near-infrared ultra short laser pulse is focused into the gas target in the vacuum cavity through a lens, and the strong nonlinear interaction between the light and the electrons inside the gas atoms leads to the generation of coherent extreme ultraviolet light pulses. Usually, in order to generate these high-order harmonics, the intensity range of the incident laser pulse interacting with inert gas atoms is 1013-1015 W/cm2.
[6] English abbreviation HHG: High Harmonic Generation
[7] In 1993, while conducting postdoctoral research at the Laser Optoelectronics Center at the University of Laval in Canada, I met a friend from Nankai University whose doctoral thesis topic was on the generation of high-order harmonics.
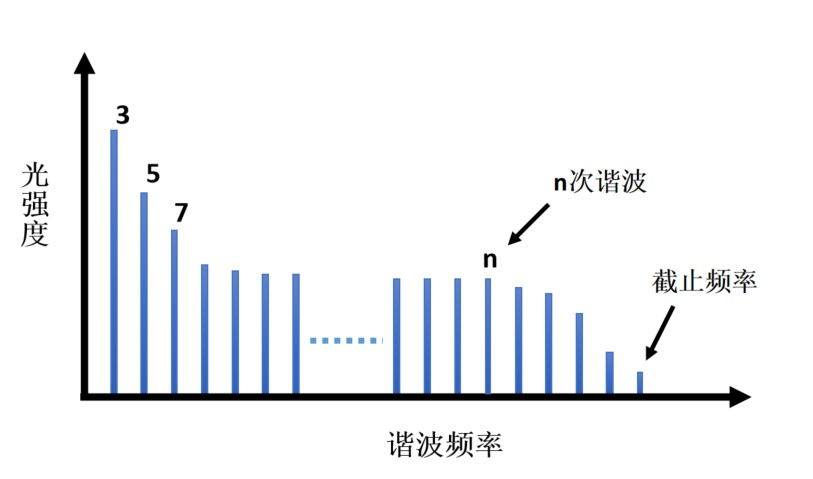
Schematic diagram of high-order harmonic spectrum generated by ultra short and ultra strong laser pulses in vacuum gas targets
It is worth noting that, unlike the commonly used nonlinear optical methods in the laser industry, which generate 2nd, 3rd, 4th, and even 5th harmonic waves in nonlinear crystals through frequency doubling (or combining) techniques, the high-order harmonics generated by focusing ultra short and ultra strong laser pulses into atomic gases include many orders of harmonics at least 3rd [8], and their highest order harmonics can even reach hundreds of orders, The shortest XUV wavelength can reach several nanometers. For example, assuming the center wavelength of the incident near-infrared light pulse is 750 nanometers, the center wavelength of its 101st harmonic is only 7.4 nanometers! In fact, this is already in the X-ray band. (The coherent superposition of higher-order harmonics gives a time-domain attosecond pulse sequence, and the interval between adjacent pulses is half of the period of the incident light pulse.)
[8] Due to the inversion symmetry of gas media, high-order harmonics in gases are generally only generated by odd order harmonics.
From the schematic diagram of the high-order harmonic spectrum, it can be seen that as the harmonic order increases, the intensity of the initial few harmonic orders monotonically decreases, followed by a relatively wide flat region. In a flat area, the intensity of harmonics decreases very slowly with the increase of harmonic order. Near a certain harmonic order at the end of the flat area, the intensity of harmonics rapidly decreases and a cutoff occurs. Based on the analysis of quantum theory, the energy of harmonic photons at the flat cutoff of high-order harmonic spectra follows the formula Ecutoff=Ip+3.17Up. Among them, Ecutoff= ω C ℏ, ω C is the cutoff frequency (corresponding to the cutoff wavelength of higher-order harmonics), ℏ is the Planck constant, Ip is the atomic ionization energy, and Up is the vibrational energy of electrons driven by a light field, also known as (existing) mass kinetic energy. The mass and kinetic energy are related to the peak intensity and wavelength of the incident laser. For a given light intensity, a long wavelength incident light pulse acting on a high ionization energy atomic gas medium can generate greater mass and kinetic energy, resulting in higher order harmonics. In addition, gases with higher ionization energy Ip can produce higher levels of harmonics. Therefore, the extension length (or maximum harmonic level) of the high-order harmonic flat region is related to parameters such as the type of gas medium used, the intensity of the incident laser pulse, and the center wavelength of the laser.
According to the principle of Fourier transform, the higher the order of higher-order harmonics (i.e. the shorter the wavelength), the wider the spectral range covered by harmonics, and the shorter the width of the corresponding time-domain pulse under precise control of harmonic phase. As shown in the figure below, when the light pulse is very short, the vibration period of the electromagnetic field contained within the pulse width will correspondingly decrease, at least as little as one or two light wave periods. The width of one cycle of electromagnetic oscillation is equal to the wavelength divided by the speed of light. For example, the electric field vibration period of 750 nanometer wavelength light is 2.5 femtoseconds, while the electric field vibration period of 7.5 nanometer wavelength XUV light is only 25 attoseconds. This also explains from another perspective the fundamental reason why the central wavelength of the attosecond light pulse falls in the extreme ultraviolet band of the XUV.
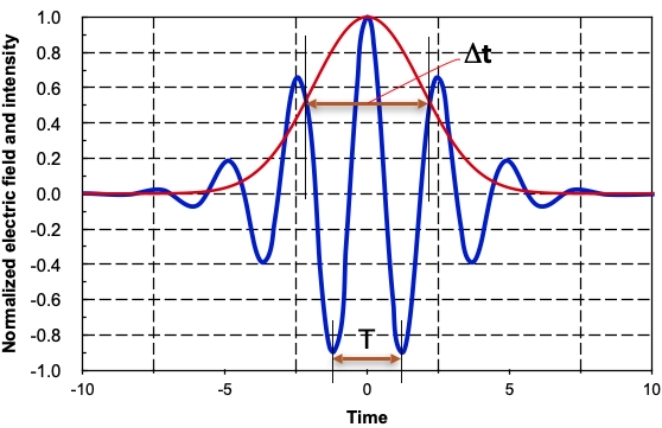
The light pulse electric field (blue) and light intensity (red) with a pulse width slightly smaller than two optical vibration periods. It is obvious that for such light pulses, the shorter the light wave period T, the wider the pulse width Δ The smaller the t.
Because the wavelength corresponding to a vibration period of 1 femtosecond in the optical field is 300nm, for a dual period pulse,To make its overall pulse width less than 1 femtosecond, its optical wavelength must be less than 150nm
Microscopic physical model of attosecond light pulse generation
The coherent superposition of high-order harmonics to generate attosecond optical pulses is only a phenomenological explanation starting from the frequency domain. In order to gain a deeper understanding of the process of generating attosecond light pulses, it is necessary to consider the electron dynamics within gas atoms under the influence of the incident light field. Describe the microscopic physical mechanism that generates XUV radiation from the time domain. The physical process of producing high-order harmonic radiation by atoms located in a strong field can be briefly described as the following three steps:
1) Under the action of a strong laser electric field, atoms experience distortion in their original Coulomb field, symmetry is disrupted, and ionization occurs;
2) The quasi free electrons generated by ionization that break free from the bound state gain additional kinetic energy under the action of an oscillating external field, accelerating their return to their parent nucleus;
3) The high-speed moving electrons that gain additional kinetic energy collide with the atomic nucleus, and at the moment when they recombine to the ground state in their vicinity, they emit XUV photons. (The energy of XUV photons is equal to the sum of the ionization energy of atoms and the energy obtained by electrons from the laser field.)
Furthermore, it is closely related to the instantaneous intensity of the incident laser electric field, and the ionization mechanism of a single atom under the action of a strong laser field can be divided into three different types. They are:
(a) Multiphoton ionization: When the laser intensity is below 1014 W/cm2, atoms or molecules absorb multiple photons at the same time and quickly ionize beyond the ionization threshold, which is called multiphoton ionization. Generally, quantum perturbation theory can analyze multiphoton ionization, which is applicable to relatively low incident light intensities.
(b) Tunnel ionization: If the ionization energy of an atom is Ip, that is, the energy of electrons in that atomic state is - Ip, which is lower than the atomic barrier height, ionization is not allowed in classical mechanics. However, in reality, due to quantum mechanical effects, electrons will pass through potential barriers with a certain probability, causing atoms to ionize, which is known as tunneling or tunneling ionization. When the laser intensity approaches or slightly exceeds 1014 W/cm2, the ionization of atoms is mainly tunnel ionization.
(c) Above threshold ionization, also known as over potential barrier ionization. When the laser intensity exceeds 1015 W/cm2, under the action of its photoelectric field, the Coulomb potential of atoms undergoes severe distortion, so that the height of the distorted potential barrier is lower than the ionization energy Ip. Electrons in the bound state of atoms have a high chance of directly crossing the atomic potential barrier, causing ionization. In this case, the ionization rate of atoms is very high, and the ionization probability is close to 100%.
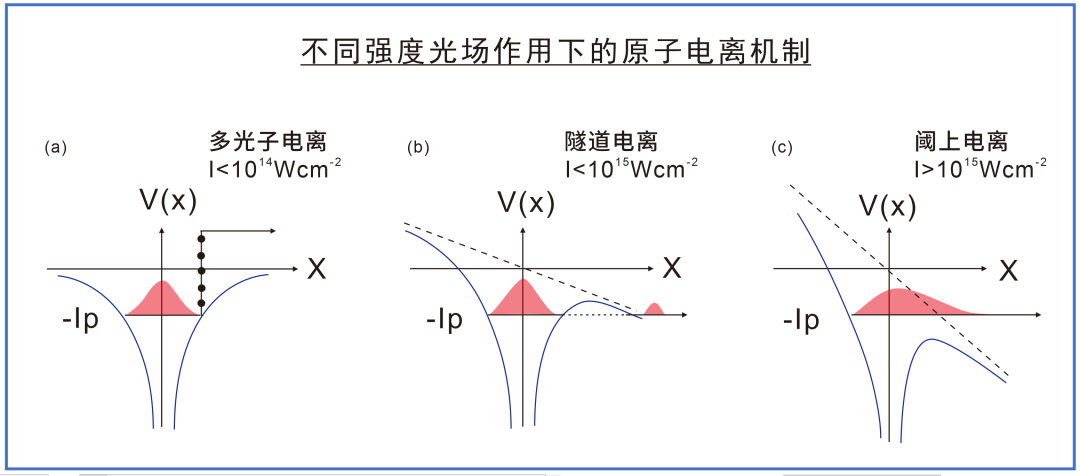
The atomic ionization and high-order harmonic generation under the action of transient strong light fields are actually two aspects of the same physical phenomenon. They are also the core of understanding the generation and measurement principles of attosecond light pulses. For readers who wish to gain a deeper understanding of the field of nanosecond science and technology, they can download "advanced physics prize2023. pdf" from the website of the Nobel Prize Committee.
For laser professionals seeking deep understanding, it is recommended to refer to Ferenc Krausz and Misha Ivanov's "Atosecond physics", Rev Mon Phys 81, 164-221 (2009).
A Brief History of Generating Ultra Short Pulse Laser
Let's briefly review the process of generating ultra short pulse lasers.
Since the first appearance of lasers in 1960, researchers have been seeking to generate laser pulses with increasingly shorter durations. Early efforts included obtaining Q-switched giant pulse output by suddenly changing the optical loss inside the laser cavity, with an output pulse width in the nanosecond range. In 1964, with the emergence of laser mode-locked technology, pulsed laser output broke through the nanosecond scale and entered the picosecond range. During this development process, dye lasers based on broadband passive mode-locked technology have continuously created records of the shortest pulse output. With the deepening development of pulse chirp compensation technology, researchers broke through the picosecond limit in the late 1970s and advanced the pulse width to the sub picosecond level. In 1981, the proposal of collision mode locking technology in dye lasers brought the study of ultrafast phenomena to the femtosecond scale. Afterwards, the chirp compensation technology was further improved, resulting in the shortest output of the dye laser reaching 27 femtoseconds and a record breaking external compression of 6 femtoseconds.
In the early 1990s, titanium doped sapphire lasers based on Kerr lens effect were born, advancing femtosecond pulse mode-locked technology to a new level. In addition to further shortening the pulse width, from less than 20 femtoseconds to less than 10 femtoseconds, the record of the shortest laser pulse width is further refreshed. More importantly, the pulse energy, pulse stability, and even the average power of femtosecond laser are significantly improved. Through strict dispersion management and optimization control, the pulse width output directly from the titanium sapphire oscillator ultimately reaches 4.5 femtoseconds. At the same time, this technological approach has come to an end for obtaining shorter sub femtosecond or attosecond pulses. Because lasers in the visible and infrared regions no longer provide the spectral bandwidth required to obtain sub femtosecond pulses [9].
[9] The 4.5 femtosecond optical pulse in the near-infrared band contains less than two periods of optical field vibration. In this band, even a single period of light pulse has a width of about 2.5 femtoseconds.
强场作用下气体高次谐波的产生有效提供了向短波长延伸的光谱极宽的相干辐射,为突破飞秒脉宽极限提供了可能性。2001年,在法国萨克雷研究中心从事强场作用下气体电离研究多年的Pierre Agostini,与其团队采用双色-双光子电离的检测方法,率先证实了高次谐波彼此之间相位锁定,导致了脉宽为250阿秒的脉冲序列的产生(Science 292, 1689 (2001))。同年,由奥地利维也纳大学(Ferenc Krausz等)、加拿大国家研究院斯泰西分子科学研究所(Paul Corkum)和德国比利菲尔德大学科研人员组成的国际研究小组,在实验中采用光谱滤波法和互相关测量手段首次产生了中心波长在14纳米、脉宽600阿秒单个软X射线脉冲(Nature 414, 509-13(2001))。这些开创性的研究成就标示了人类在时间尺度的操控上已步入阿秒时代[10].
[10] In 2017, a research group from the Swiss Federal Institute of Technology generated 43 attosecond light pulses (Optics Express. 2527506 (2017)). In 2023, a research team from the University of Rostock in Germany generated electron pulses with a time width of only 53 nanoseconds (Nature 613662 (2023)).
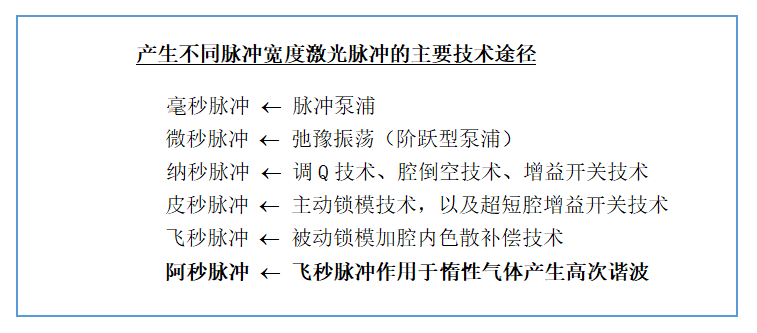
值得强调的是,除阿秒光脉冲外,其它时间宽度的脉冲,从毫秒到飞秒,都是可以直接从激光器产生的。而阿秒光脉冲却不是。要产生阿秒光脉冲,必须要先有高质量的飞秒光脉冲,这是必要条件。此处的高质量主要指的是高稳定性、脉宽足够短、光束空间特性接近衍射极限,和有足够大的单脉冲能量。而后者则需要用到,或者说得益于啁啾脉冲放大(CPA)技术。CPA(即Chirped Pulse Amplification)技术是将入射飞秒(或皮秒) 脉冲先展宽,然后再放大,最后再进行脉宽压缩。这样能充分避免光放大过程中的非线性效应,进而在不损坏光学增益介质的前提下,有效地提高光脉冲能量和峰值功率。由于欲在气体内产生高次谐波,入射光场必须足够强,因此,CPA技术的娴熟运用对阿秒光脉冲产生也是至为重要的[11].
[11] Introduction to the 2018 Nobel Prize in Physics:
https://www.nobelprize.org/prizes/physics/2018/summary/
下图给出不同宽度光脉冲的主要测量方法。其中用于飞秒光脉冲测量的“频率分辨光学门”与“频率剪切干涉”这两种法,应该均可以拓展至阿秒脉冲的测量。它们与双光子干涉阿秒拍频重建法和光场驱动电子条纹相机实际上是殊途同归,最终都是得到光脉冲的电场与相位分布。而图中列出的其余几种测量方法,除自相关法可包含部分相位信息外,所测的都只是脉冲功率或光强分布,即振幅包络的平方值.
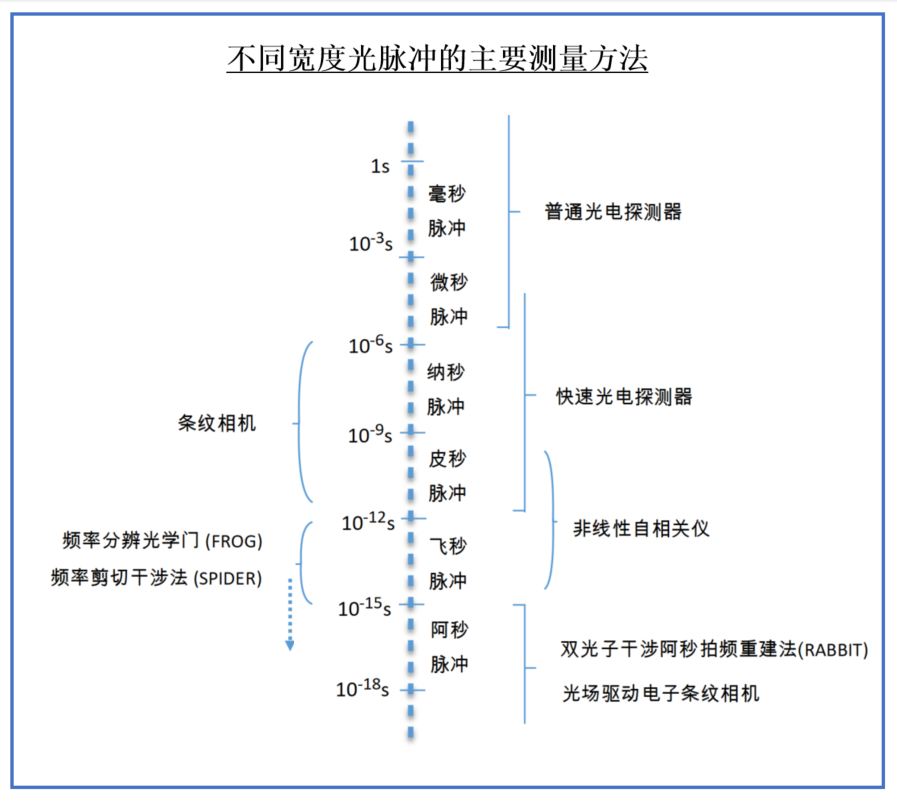
The generation of a single attosecond light pulse
下图所示为双周期近红外飞秒脉冲作用下产生软X-射线阿秒单脉冲的主要原理和基本步骤。即首先(在He气中)产生高次谐波,而后通过(重元素Zr作为)滤波器选取单个阿秒脉冲,接下来,再在Kr气靶中进行红外飞秒脉冲与软X-射线阿秒脉冲的互相关测量.
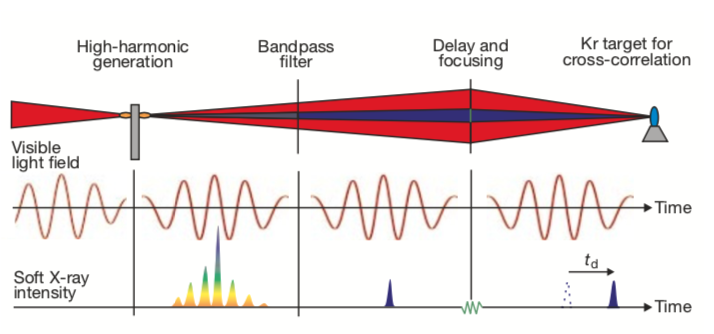
Image from Nature 414509-13 (2001)
Based on the in-depth analysis of the ionization process under strong field effects introduced above, we can obtain two very important characteristics for generating attosecond XUV light pulses:
1) Within each driving light field cycle, two attosecond pulses are generated, corresponding to the collision of the accelerated electron with the atomic nucleus in two different directions, which is also the moment when the maximum kinetic energy of the two electrons is obtained;
2)每一个阿秒XUV脉冲都是能量或频率啁啾脉冲,也就是说,沿时间轴阿秒脉冲的不同点位对应的频率不同。这为采用光谱滤波器获得单个阿秒脉冲提供了可行性。(这也正是上图中最下方一行图示的情况).
What is the purpose of attosecond light pulses
简单地说,由于空间尺度与时间尺度间的内在关联性,原子内电子壳层的特征动力学时间尺度大都处在阿秒时间范围[12]。例如,氢原子基态的电子轨道运动周期为152阿秒,光电效应中电子飞离原子核的时间也仅有几十阿秒量级。因此,借助于阿秒光脉冲,特别是单个阿秒脉冲作为超高时间分辨的探针,科学家们将能够采用类似密集快速采样的方式观察记录原子、分子中电子的运动过程,从而在物理学、化学、生物学、材料科学等诸多领域内微观现象研究中开辟出崭新的科学探索天地.
[12]我们知道,空间尺度通过速度与时间联系起来。而根据牛顿定律越微小的物体越容易被加速。由经典量子力学不难估算出,氢原子内基态电子的轨道速度接近光速的百分之一。如以这样的速度跨越0.05纳米大小的原子空间,所需时间仅为20几个阿秒.
我们知道,在教科书或科普读物中常见的、如下图所示的原子、分子中的电子运动轨道或电子云模型,实际上都仅是基于量子理论的预测而得到的,从未从实验上测量验证过。这是因为原子周边电子运动的空间实在太小、速度实在太快了,需要有非常短暂的时间探针才可能去分辨其运动过程。而阿秒光脉冲则预期可以用来面对这一经久未决的技术挑战.
阿秒光脉冲将为验证量子理论,开展各种量子现象、量子效应的实验研究,尤其是涉及原子尺度内电子运动时间特性的研究,打开一扇新窗。基于阿秒光脉冲的阿秒光谱计量学、阿秒物理学、阿秒化学、阿秒生物学等,将会构成阿秒科学新篇章。不难预计,阿秒科学必将会使得人类真正在原子尺度上实现高分辨时间测量,将使得对自然界中超快现象、超快过程的观测范围拓展到各种物质形态内电子运动过程的梦想成真.
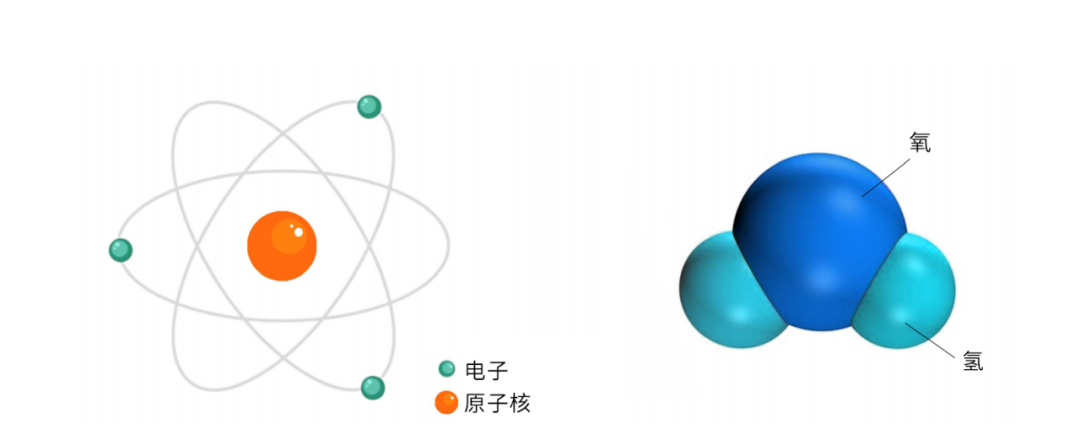
Models of electron orbital motion and electron cloud in atoms and molecules
另外,值得补充的是,因为阿秒光脉冲的波长处在极紫外波段,所以其本身便是一种独特的脉冲X射线光源。换言之,阿秒光脉冲技术的发展为开发性能优越的脉冲X射线光源提供了一个全新的路径[13].
[13]为国人关注的光刻机的光源即在极紫外。或许在不远的将来阿秒XUV光脉冲在光刻上也能发挥作用,尤其是在降低光刻机造价和对系统稳定性要求方面。当然这要求阿秒XUV光脉冲的能量要能达到足够高.
总之,以阿秒XUV光脉冲产生、阿秒光波电子学为特征的阿秒科学的诞生,使得一直以来便引人瞩目的超短脉冲激光、超快速现象、超强光与物质相互作用的研究跨入到一个新的里程碑。它也为今后迈向子秒(10-21s)这样更短的时间尺度,从而实际分辨与观测原子核内部粒子运动打下重要的基础[14]。可以预计, 阿秒科学必然为未来科技进步,尤其是基础科学的研究发展开拓广阔的可能性.
[14]可以推测比阿秒更短的时间尺度子秒(10-21s,又称仄秒)将是原子核内粒子运动的特征时间,并与更高能量或更高频率的伽马射线辐射的动态过程密切相关.
Finally, let me share with you some highlights about the current Nobel Prize in Physics:
1) The Nobel Prize Committee contacted Anne L'Huillier multiple times, but no one answered the phone because she was teaching her students at the time. (I hope that in the near future, teachers from a certain university in China will also receive a call from the Nobel Prize Committee when giving lectures to students.)
2) People have probably heard the saying "the 20th century is the era of electrons, and the 21st century is the era of photons.". The direct implication is that in the 21st century, the driving force of electrons on scientific and technological development will be more replaced by photons. But Nobel laureate Dr. Ferenc Krausz from the Planck Institute for Quantum Optics in Germany does not agree with this viewpoint. In Krausz's view, human observation and manipulation of electron motion processes at the atomic scale have only just begun. (This kind of cognition can be attributed to the birth of attosecond light pulse technology, or it can be considered as a driving force for his own research on attosecond light pulses.)
3)网上有人称L’Huillier是“阿秒之母”。而如果问ChatGPT谁是“阿秒之父”的话,它会告述你,被誉为“阿秒之父”的正是提出强场作用下电子散射-高次谐波产生三步模型的加拿大国家科学院的Paul Corkum博士。但Corkum此次却未能获得诺奖。(这着实让一些人感到了意外和为之遗憾)。这有可能出自两个原因,一是颁奖理由中的关键词是产生阿秒光脉冲的实验方法(从某种意义上来说,本年度的诺贝尔物理奖所奖励的,一方面是一项重要科学门类的发展及打开未知科学领域的新发现,另一方面则更侧重于基础性科学实验能力的突破。);二是最早独立提出、解释高次谐波产生现象的强场作用下电离模型的科学家,还包括美国劳伦斯利乌莫儿国家实验室的K.C. Kulander[15]。阿秒光脉冲的产生、阿秒科学的发展历程,也充分体现了国际上不同研究组之间的科学合作与竞争.
[15] Kulander团队与Corkum各自的关键性研究论文同在1993年的美国《物理评论快报》上发表,但Kulander团队的论文发表在三月,而Corkum的论文在九月.